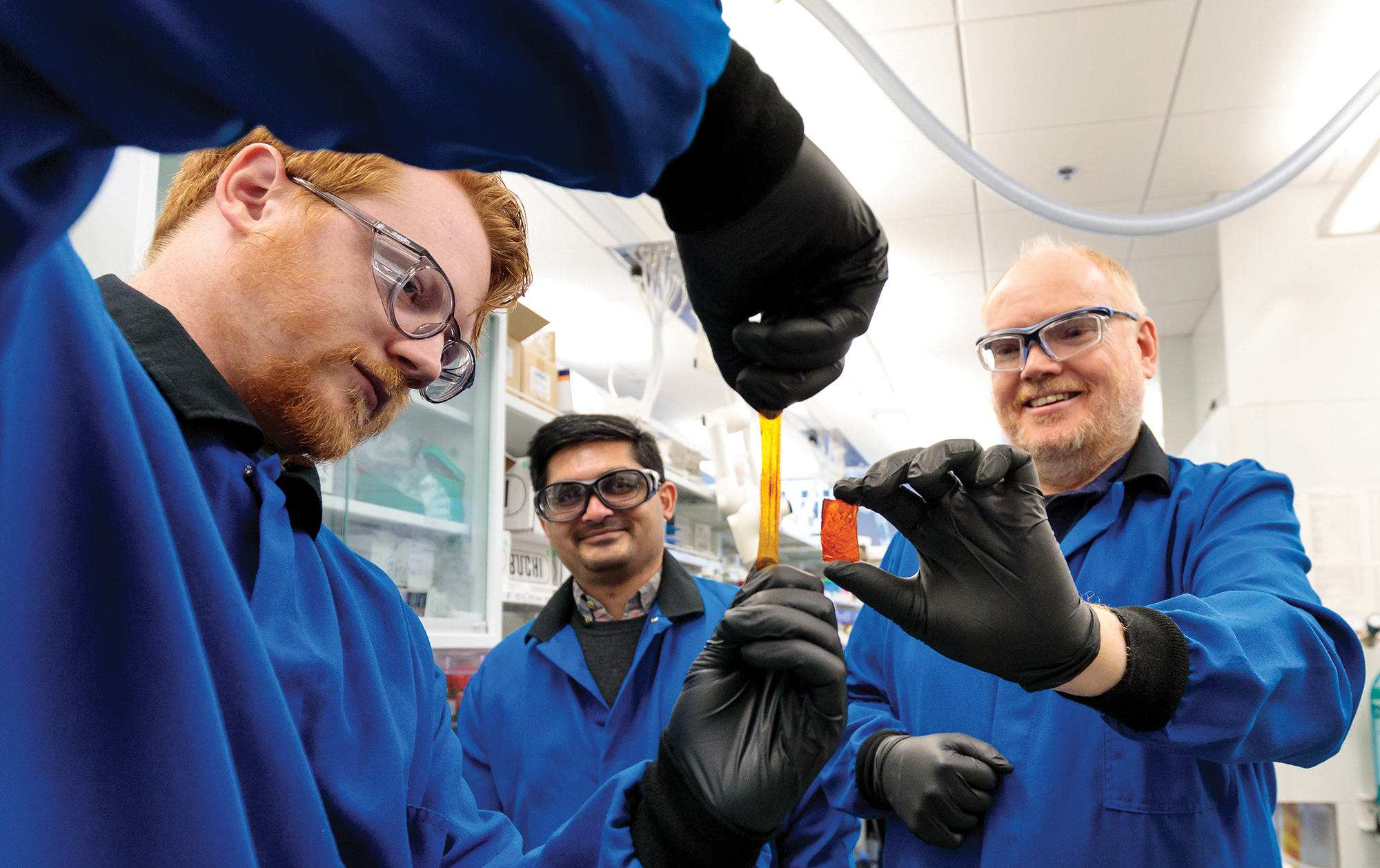
From left: Nicholas Boynton, Shrayesh Patel, and Stuart Rowan with their pluripotent plastic material, which can be tempered to take many forms. (Photography by John Zich)
They flex, glow, filter, and shape-shift—for the greater good. Meet the futuristic new materials developed by UChicago scientists that could soon be all around us.
There’s an intuitive appeal to materials science. “It is easy to get a fourth grader interested in materials science, as materials such as plastics and metals impact almost every part of daily life,” says Stuart Rowan, Barry L. MacLean Professor for Molecular Engineering Innovation and Enterprise in the Pritzker School of Molecular Engineering (PME). After all, when you’re stretching and squishing a ball of Silly Putty, polymers start to seem pretty exciting. “It’s very tactile in that way.”
The University of Chicago has long been a leader in this stretchy, squishy, and intriguing field. The Materials Research Science and Engineering Center, which Rowan leads, was founded in 1961. In those early days, physicists and chemists were “applying their skill sets to materials in really unique ways—and really pushing the boundaries of what could be done,” Rowan says. The arrival of molecular engineering at UChicago brought a new group of scholars keenly interested in making materials to solve real-world problems related to water, energy, health, sustainability, and more. Today research focused on the underlying science of materials is complemented by a new emphasis on application and impact. That range of interests and approaches, Rowan says, has created a strong intellectual community: “Like anything, if you bring really smart people together, fun stuff is going to happen.”
The sense of camaraderie “is really important, not just for me, but for my students,” says Sihong Wang, an assistant professor in PME who makes stretchable bioelectronics (see below). “It’s impossible for one person or one group to know everything. Having a local community with complementary expertise is really empowering for what you can do and what you can think about.”
Here are just a few of the new materials that scientists including Rowan and Wang have dreamed up—and made real.
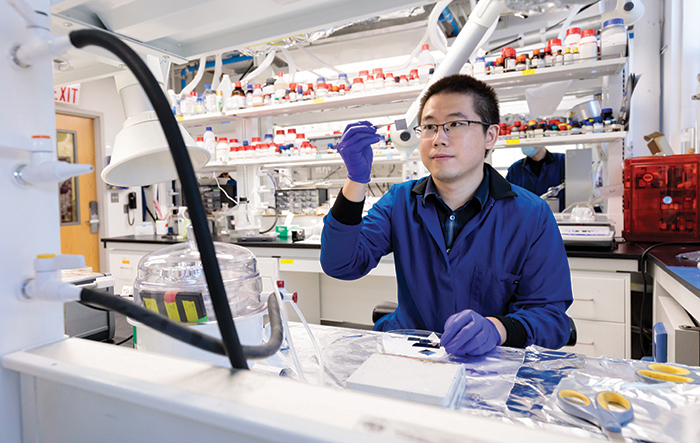
The material: A stretchable organic light-emitting diode (OLED) display
A leap forward: Today’s wearable health monitoring devices, such as Fitbits and Apple Watches, are limited in the information they can capture, because they don’t have continuous contact with the body—even subtle movement creates small gaps between the device and the skin. “That gapping is creating a huge problem,” explains Sihong Wang. Without close contact, the devices struggle to accurately record information such as heart rate.
But what if you could create a health monitor whose screen moves with the wearer? That’s the motivation for Wang’s material, a stretchable OLED display that he designed alongside Juan de Pablo, Liew Family Professor of Molecular Engineering and executive vice president for Science, Innovation, National Laboratories, and Global Initiatives.
The big picture: “Overall, what we are working on is trying to bring electronic devices much closer to, and make them more interactive with, the human body,” Wang says.
The challenge: OLED displays are made up of small organic compounds that sit between two electrodes and emit light when stimulated by electricity. The materials used in today’s OLED TVs and smartphone screens are highly rigid, but scientists have been experimenting with bendable versions since 2011. Wang and de Pablo’s design involves a stretchable polymer that can convert electrical energy into light extremely efficiently through a process called thermally activated delayed fluorescence.
Big stretch: The new material can bend in half and stretch to twice its original length without losing the ability to display a clear image.
Next steps: Wang says the material needs refinement before it will be ready for commercialization and large-scale manufacturing. But he hopes in the coming decades it could be used in wearable devices with the ability to accurately and unobtrusively monitor health information like blood pressure, heart rate, and oxygen saturation.
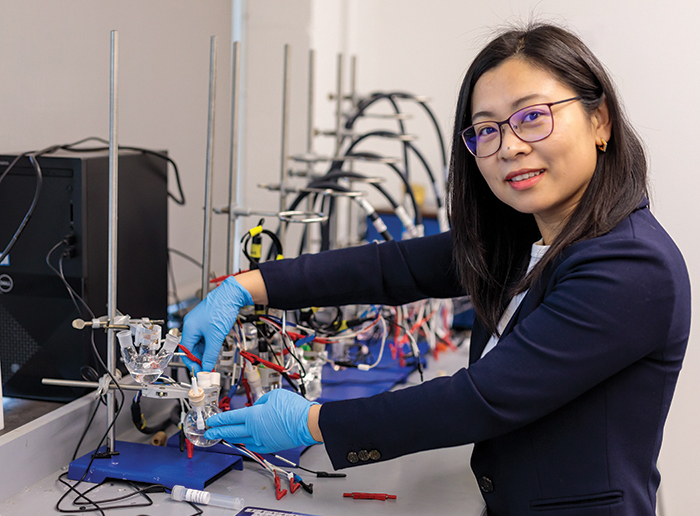
The material: An electrochemical lithium “trap”
The big picture: Lithium-ion batteries power everything from smartwatches to electric cars. That’s created a massive demand for the light silvery metal. Today much of the world’s lithium comes from Chile, Argentina, and Bolivia, where it is abundant in salty groundwater. To extract the lithium, miners pump out the saltwater, sequester it in ponds, and allow it to evaporate, leaving the lithium behind—a process that can take almost a year. In addition to being slow, brine mining is environmentally costly: the process requires immense amounts of water, as well as chemicals that can contaminate the local soil and water supply.
Hoping to sidestep those challenges, scientists such as Chong Liu, Neubauer Family Assistant Professor of Molecular Engineering, are searching for alternative methods of harvesting lithium from saltwater. Liu’s ultimate goal? To develop a material sensitive enough to capture the plentiful but extremely dilute lithium from the world’s oceans.
No sodium allowed: Submerging lithium-ion electrodes in saltwater could be an efficient and safe means of lithium extraction. But lithium-attracting electrode materials have a nasty habit of also trapping sodium, lithium’s downstairs neighbor on the periodic table. Liu’s group uncovered how lithium and sodium compete in olivine iron phosphate materials and designed strategies to promote lithium during the competition.
The advantages: “Our big motivation is to create a process that’s as environmentally friendly as possible,” Liu explained in 2021. “Since we are taking an electrochemical approach, we completely avoid the need for intense heat or strong acids, and we get only the element we want.”
Next steps: Liu has continued to refine the new approach to lithium extraction; in a 2022 paper, for example, she and her team discovered that “seeding” an electrode with lithium ions could help keep pesky unwanted elements away.

The material: A building coating that can heat or cool
The big picture: Keeping indoor spaces comfortable comes at a major financial and environmental cost. Heating and cooling buildings accounts for around 15 percent of all energy use in the United States. In a warming world, experts fear, the demand for air-conditioning—and the associated greenhouse gas emissions—will only increase. Po-Chun Hsu, an assistant professor in PME, hopes to prevent that outcome by developing new materials that allow for “thermal comfort,” as scientists call it, with a smaller energy footprint. To that end, Hsu and colleagues designed a building coating that can trap heat or release it, depending on the temperature outside.
Think of it like … : “We’ve essentially figured out a low-energy way to treat a building like a person; you add a layer when you’re cold and take off a layer when you’re hot,” Hsu explained in 2023.
Chameleonic coating: When it hits any selected trigger temperature, the ultrathin film can switch easily between heating and cooling modes—thanks to a layer of copper that changes from a heat-absorbing solid to a heat-emitting liquid with a shock from an electrode made of graphene. In cooling mode, the material can emit up to 92 percent of its absorbed infrared heat, the invisible heat given off by all people and objects; in heating mode, it emits just 7 percent, absorbing the rest to help keep the coated building toasty inside.
Savings and costs: The researchers estimate that the new material would reduce the energy consumption of a typical building’s climate control system by 8.4 percent each year. It also requires very little energy to operate—less than 0.2 percent of the total energy use of a typical commercial building. However, an important barrier to widespread use of the material, at least right now, is cost: copper and graphene don’t come cheap, so Hsu’s team is experimenting with less expensive alternatives.
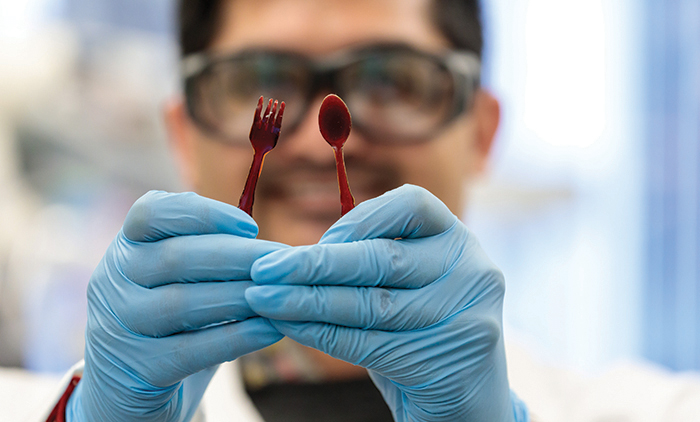
The material: Pluripotent plastic
The inspiration: “I’m always incredibly jealous about how good biology is,” says Stuart Rowan. “In this case, I was super jealous of pluripotent stem cells,” which can become almost any type of cell in the body. Plastics, by contrast, tend to have circumscribed properties and therefore applications: the material used to manufacture a milk jug, for example, couldn’t be used to make cling film. Rowan had long been intrigued by the challenge of developing a single material that could serve the many and varied purposes fulfilled by modern plastics.
In 2023—along with collaborators including Shrayesh Patel, an assistant professor in PME, and PhD student Nicholas Boynton—Rowan published a paper describing a material that can be tempered and retempered to take on multiple forms. When heated to about 140°F, the plastic becomes a stiff, high-strength material; raise the temperature even higher, to about 230°F, and it becomes soft and flexible and can be used as an adhesive.
Beakthrough: Most plastics are made from long polymer chains held together by covalent bonds; if those bonds are broken, the material degrades. Rowan and his collaborators designed a material united by a particular type of covalent bond that can be easily broken and re-formed.
Think of it like … : “I always think of the blacksmiths that you see in the movies,” Rowan says. “The temperature of the fire dictates the properties of the steel that you get.”
Challenge accepted: In the manuscript they submitted for publication, Rowan and his coauthors wrote that they believed their material was stiff enough, in its hardened form, to be used as an eating utensil. A peer reviewer encouraged the team to put their claim to the test, so Boynton made a pluripotent plastic spoon and used it to scoop peanut butter from a jar. A video of this feat appears as a supplement to the paper.
The advantages: The pluripotent material is well suited to resource-scarce environments—think of remote fieldwork or even space travel—where you can, through tempering, have on-demand material properties. And though it could be taken to another planet, the pluripotent plastic is also better for ours, because it can be recycled much more efficiently. Today “you need to separate plastics if you’re going to recycle them,” Rowan explains. But if more items were made from a single feedstock of pluripotent plastic, it would be easier to combine and reuse them.
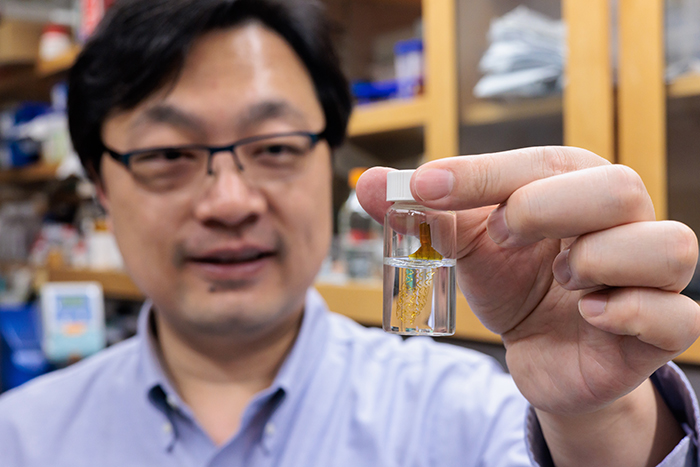
The material: A springy, microscopic “soil”
The inspiration: Soil, a seemingly humble material with a surprisingly rich set of behaviors. With the addition of light, water, or pressure, it can assume new forms—all of which affect the many tiny organisms who call it home. Taking cues from the ground beneath our feet, professor of chemistry Bozhi Tian and his colleagues got their hands dirty, devising a soil-like material designed to promote microbial growth. The material is made up of starch, tiny particles of clay minerals called nanoclay, and liquid metals.
Think of it like … : The new material, like the one that inspired it, is highly porous. This open structure, with its abundant nooks and crannies, creates an ideal environment for microbes to move in and flourish. “We found the porosity is very important; we call it the partitioning effect,” Tian said in 2022. “I think of it like a meeting—if you break a large meeting or class into smaller sections, there will be more interaction.”
Secret sauce: Though the researchers don’t yet know exactly why, the inclusion of liquid metal appears to be particularly important for microbial growth; without it, prototype versions of the material were less successful at fostering bacterial growth.
Gut feeling: Tian and his colleagues believe their ultra-high-tech “soil” has a range of potential applications. One is the treatment of digestive diseases thought to arise from a lack of gut microbe diversity. The researchers tested this theory in mice; a small dose of the material reduced the severity of colitis symptoms. The material could also speed up the fermentation that creates biofuels like ethanol.
Further afield: Tian hopes to develop other environmentally inspired materials. “Soil is just the beginning,” he said. “There are many ways we can learn from nature.”