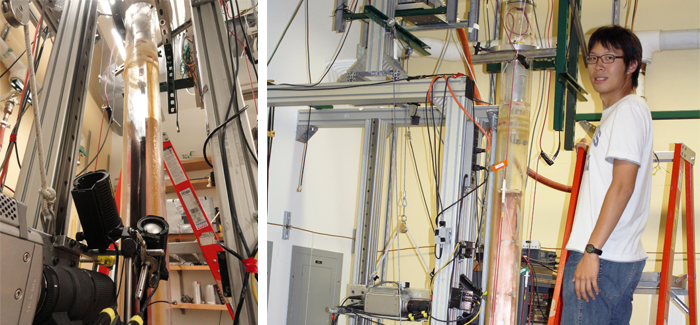
Jaeger’s experimental setup includes a camera that drops in near free fall with the charged grains, capturing their intractions as they drop through an airless tube. (Photography by Victor Lee)
What scientists don’t know about static electricity might shock you.
Many children’s first physics lesson is in static electricity—when they shock themselves on a doorknob after shuffling their stocking feet on carpet. But even children who grow up to be physicists don’t understand exactly how static charges come about. “This is one of the most vexing yet unresolved problems that we have right now in materials science,” says Heinrich Jaeger, the William J. Friedman and Alicia Townsend Professor in Physics. Now Jaeger and his research group have performed an experiment that might shed some light on that mystery.
The conventional theory describing static electricity states that when two dissimilar materials rub together, one transfers electrons to the other and both develop an electrical charge. The size of this charge depends on the materials used, but one will always lose charge and the other will always gain it.
What if the two pieces of material are identical? The theory predicts that, having the same properties, the two pieces should exchange equal numbers of electrons, and therefore neither should end up charged. But that’s at odds with experimental evidence.
Jaeger’s work has focused on insulating grains (static charges on metals are better understood). Insulating grains are technically solids but under certain conditions also have liquid-like properties. Sand, for instance, is solid enough that you can stand on a pile of it, but it flows through your fingers like water.
Part of Jaeger’s research is to determine which of these materials’ behaviors are solely due to mechanical forces, such as when the grains bounce off each other like billiard balls, and which are due to other forces, like static electricity. The static force may be small, but it carries big implications for any scientific or industrial process that depends on manipulating grains.
Five years ago Jaeger set out to test an alternative theory developed by Daniel Lacks at Case Western Reserve University: that electrons get transferred from large grains to smaller ones. Jaeger; Scott Waitukaitis, PhD’13; and Victor Lee used a 2.5-meter-long tube, emptied of air to cancel out any effect from air turbulence. The researchers dropped grains of zirconium dioxide-silicate (a nonconducting substance that acquires static charge well) in two different sizes through an opening at the top. A high-speed camera, falling at the same rate, recorded each individual interaction between grains. An electric field in the tube separated positively charged grains from negatively charged ones.
Next the researchers tried to measure how much charge actually existed on the surface of the grains in the first place. They gently heated an unmixed sample of the grains, which caused each of the electrons trapped on their surface to release a single photon. Collaborating with a team at the University of Illinois at Chicago, they measured each emitted photon.
The results were inconclusive. Jaeger’s team found that one aspect of Lacks’s theory was correct: electrons had been transferred from large grains to smaller ones. However, the charge available on the surface of the grains was much too small to account for all the transferred charges—just 1/100,000th of the total static charge. Another mechanism was at work.
Jaeger hypothesizes that the bulk of the static charges were transferred not by electrons but by ions, such as hydroxide—water molecules that have lost an atom of hydrogen. A one-molecule-thick layer of water coating the grains would be enough to account for the observed charge. A competing hypothesis from researchers at Northwestern University suggests the charge comes from nanometer-sized chunks of material ripped from one grain and attached to the next. At this juncture, Jaeger says, it’s too early to rule either idea in or out.
Whichever theory stands up to further investigations, a fuller understanding of tiny electric charges is important. Static charges cause small particles to form clusters, and the size of those clusters has powerful ramifications on other fields. In meteorology, clusters of volcanic ash particles can affect the formation of clouds; in astrophysics, clusters of charged dust particles are thought to be the first stage of planetary formation.
Jaeger also points out the potential impact in business: static charges have been blamed for grain silo explosions, and clusters of charged grains reduce the efficiency of the fluidized bed reactors used widely in the chemical industry.