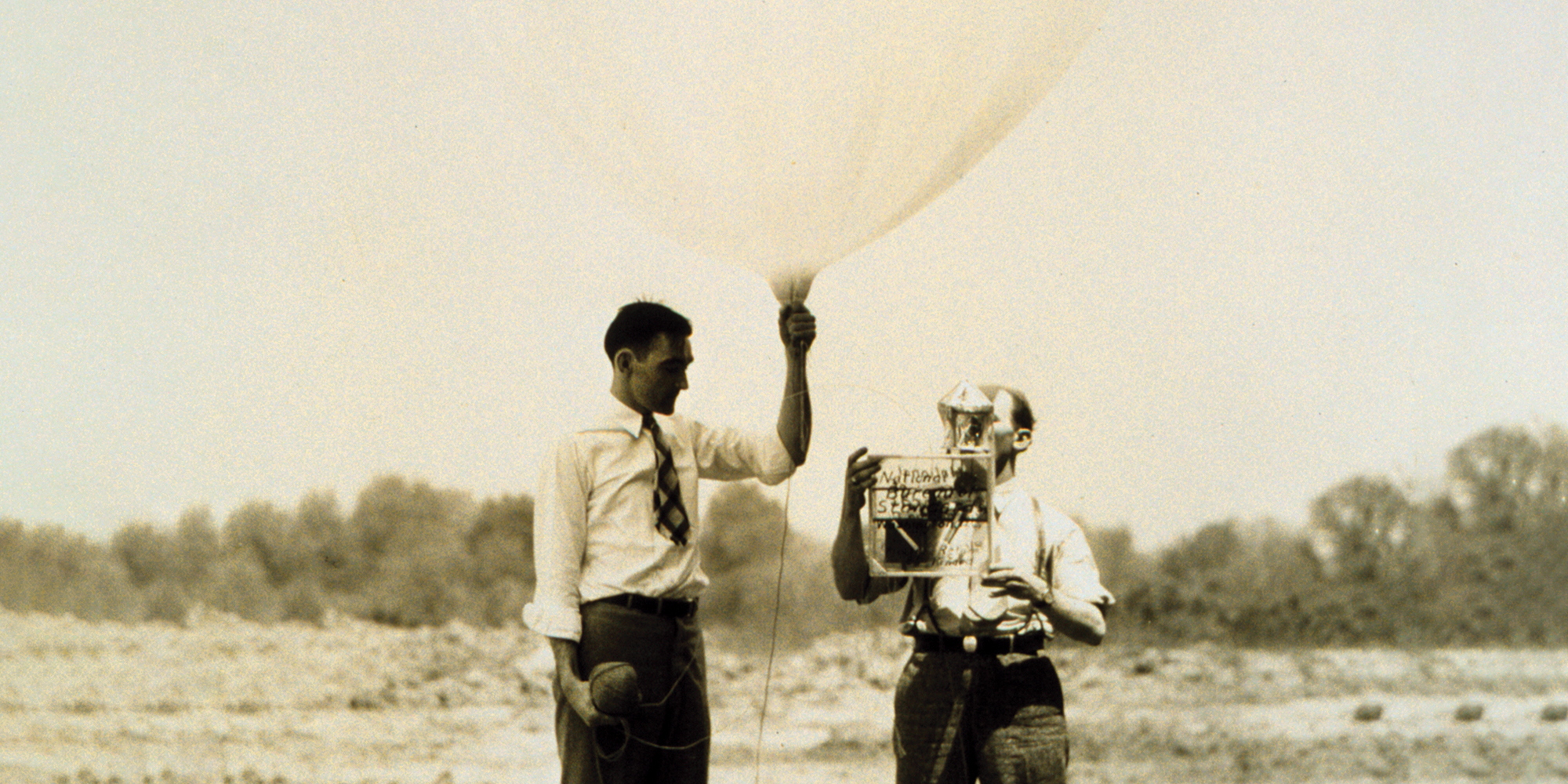
Early launch of a radiosonde—a balloon-borne instrument for taking atmospheric measurements. (Photo courtesy the National Oceanic and Atmospheric Administration)
The Chicago school of meteorology found and made waves.
At the turn of the 20th century, the United States Weather Bureau was approached by two inventors looking for a site with steady winds. Based on the forecasters’ suggestions, the Wright brothers completed the first powered airplane flight in 1903 near Kitty Hawk, North Carolina. Their famous flight marked the birth of aviation and the beginning of a fast friendship between flight and meteorology.
The two fields evolved in a symbiotic relationship. Scientists had begun to understand that upper atmosphere activity had a significant impact on surface weather, and aviation offered meteorologists a new perspective. Pilots benefited from weather forecasting for safety and efficiency, and in return, instrument-outfitted aircraft could collect meteorological data not available from the ground.
By 1940 Swedish meteorologist and émigré Carl-Gustaf Rossby was the most prominent proponent in America for investigating the upper atmosphere. Rossby led the conversion of weather research from a descriptive to a predictive science by applying the laws of physics and mathematical modeling. When he became the first head of the University of Chicago Institute of Meteorology, the appointment ushered in two crucial decades when the University helped shape the developing field. The Chicago school of meteorology forged alliances between academia, industry, and military to translate theory into practice; unlocked the mysteries of severe weather; and laid the foundation for future climate change research. UChicago’s meteorology program nominally closed its doors more than 50 years ago, but its influence continues to ripple through the geophysical sciences.
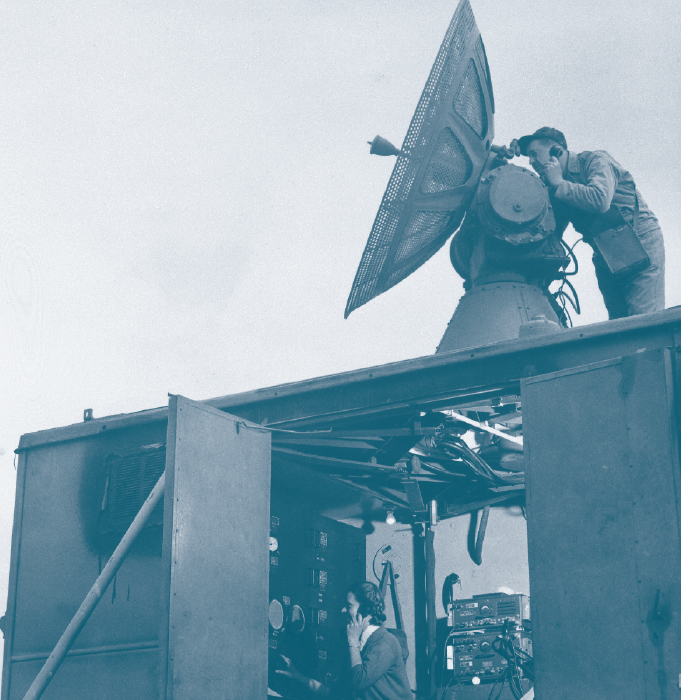
Weather forecasting can be traced back to ancient civilizations, which used astronomy to track seasonal changes. But meteorology—a subset of atmospheric science that studies weather and optical phenomena—became an empirical science in the 17th and 18th centuries, with the invention of the thermometer, barometer, and hygrometer (to measure humidity). Quantifiable data could be brought to the astronomical correlations, historical record keeping, and intuition of earlier weather forecasting.
But it took the invention of the telegraph in 1844 to merge meteorology and weather forecasting into a practical endeavor. With this technology came the ability to collect data on a systematic level and to warn people to the east—the direction that weather typically travels—that a storm was coming. The telegraph-weather marriage was proposed during the Crimean War, when a severe storm in 1854 sank French and British ships in the Black Sea. Europe implemented a storm-warning service soon after.
In America similar dots were being connected. In 1849 the Smithsonian Institution established an observation network, supplying telegraph companies with instruments to take measurements for weather maps. In 1870 the government created a national weather service, first as part of the US Army Signal Service and later becoming the civilian US Weather Bureau. For 50 years America’s weather service, and those around the world, relied on weather maps to subjectively spot patterns and forecast weather based on educated guesses.
Then in 1917 Norwegian physicist Vilhelm Bjerknes established an institute of geophysics in Bergen, Norway. There he and his colleagues brought objectivity to meteorology by infusing the field with thermodynamics and fluid mechanics, the physics of liquids and gases. Soon after the institute was founded, Carl-Gustaf Rossby left his physics program at the University of Stockholm to become a protégé of Bjerknes as the Norwegians developed the polar front theory, the foundation of the Bergen school of meteorology.
With his son Jacob, Bjerknes determined that enormous masses of cold dry air that descend from polar regions are the “leading players” in “weather drama,” wrote Time in a 1956 profile of Rossby. Their movements create the cold and warm fronts that appear in modern weather maps. Previously, large-scale rotating areas of low atmospheric pressure called cyclones, which could measure a thousand miles wide, were thought to hold top billing as they were known to bring storms. But the Bjerkneses’ discovery knocked them down to “bit-players” below the polar fronts. The development “lit up meteorology like a new sun rising and upgraded it into a more exact science.”
In 1926 Rossby, who had returned to Stockholm to finish his education in mathematical physics, won a fellowship that placed him in the US Weather Bureau to study the application of the Bergen polar front theory to American weather. During his first year in the United States, he studied turbulence and convection (the fluid-based rising of heat and sinking of cold), consulted with the booming aviation industry, and applied his mentor’s theory to US weather maps.
But the Weather Bureau wasn’t “a pleasant place for the twenty-seven-year-old Swede,” wrote Horace Byers, Rossby’s former student, longtime colleague, and biographer. “He encountered unexpected hostility to the polar-front theory and to air-mass analysis,” and clashed with the bureau’s administration over a series of minor incidents that came to a head when he made an unauthorized weather forecast for Charles Lindbergh’s flight from Washington, DC, to Mexico City. Rossby left the Weather Bureau “literally persona non grata.”
Through his involvement with aviation, Rossby connected with the Department of Aeronautical Engineering at MIT, where he established the first graduate program in meteorology in 1928. Using a research plane provided by the school and a new remote-sensing device carried by helium balloons, Rossby was able to access the upper atmosphere, where he detected the large-scale flow patterns that directly influence weather. These were planetary waves, now called Rossby waves, that naturally occur in rotating fluids. If polar air masses are weather stars, then Rossby waves are the producers.
Rossby’s research laid “the fundamental groundwork for our understanding of the mid-latitude atmosphere, between the tropics and the poles,” says Tiffany Shaw, associate professor in geophysical sciences, whose research focuses on the physics of Earth’s current and future climate. “What was beautiful about his work was that he was using a very simple model. Starting with the complicated laws of physics, he had to make assumptions and came out with this emergent property of weather waves.”
Shaw, a physicist by training, invokes the “spherical cow” metaphor to explain why physicists like Rossby tend to simplify models. The joke goes, a dairy farmer asks for help increasing milk production. A theoretical physicist replies, “I have a solution, but it only works for spherical cows in a vacuum.”
A classic approach for theoretical physicists is to simplify models for complex systems. If you want to model the motion of a thrown ball as accurately as possible, you must consider the ball’s shape, texture, surrounding air density—countless considerations that affect the physics of the trajectory. Not only does that make solving complex problems daunting, it also leads to models that accurately predict the motion of footballs thrown in high humidity, but not baseballs thrown in dry heat. But if you consider only mass, you can predict the motion, imperfectly but well enough, for different scenarios.
This is what Rossby did with his planetary waves; he modeled their motion in two dimensions instead of three, neglecting aspects such as water vapor and vertical motion. His simplified model could then be studied via atmospheric observations or laboratory experiments. Rossby’s theoretical tools, including his equations, elevated the Bergen school’s conceptual models into mathematical models, adding numerical precision to weather prediction. They also made future atmospheric science discoveries possible.
Soon after Rossby’s waves were discovered, he joined the University of Chicago to lead its brand-new institute.

The Second World War was a driving force in the founding of UChicago’s Institute of Meteorology. In early 1939, Rossby, then on the faculty of MIT, wrote to his school’s president, Karl T. Compton, to suggest the creation of a meteorology program at UChicago, where Compton’s brother was a physicist. Arthur Holly Compton brought the idea to University president Robert Maynard Hutchins and Physical Sciences Division dean Henry Gale, AB 1896, PhD 1899, but the idea was deemed financially infeasible.
Ten months later, Byers, Rossby’s mentee from MIT and colleague, independently proposed the same thing. With war having broken out in Europe, investment in meteorological research now had new importance.
Byers’s recommendation was endorsed by Gale, an astrophysicist who had served in the Signal Corps during World War I and was familiar with the Norwegian meteorological developments. Arthur Compton also championed the program, calling it an “essential part of the contribution we can make to the national defense.” He built his case for support on three points. First, the University was already conducting groundbreaking research in physics, geography, and math. Second, it favored fundamental research, and, third, it was located in a region with distinctive weather and in need of meteorologists for aviation, agriculture, and industry. The pitch was a success, and a donor gave the necessary funds to start the new program: $15,000.
UChicago joined MIT, Caltech, and New York University as the only advanced-degree granting meteorology programs in the country. (UCLA’s program, under the leadership of Jacob Bjerknes, formed around the same time.) Rossby was called to lead the institute, with Byers as his right hand.
The Institute of Meteorology opened in 1940 as part of the physics department. Its first class included 15 students from the Weather Bureau and what’s now called the Federal Aviation Administration. Rossby had envisioned a theoretical venture, but that year, President Franklin Roosevelt called for the construction of 50,000 military aircraft, requiring 10,000 meteorology-trained officers. Rossby’s academic instruction quickly pivoted to military-based cadet training.
While students left Hyde Park to go to war after Pearl Harbor was bombed in late 1941, cadets took their place. “By 1942 all available dormitory space had been consigned to military programs,” writes College dean John W. Boyer, AM’69, PhD’75, in The University of Chicago: A History (University of Chicago Press, 2015). International House was filled with meteorology cadets and Red Cross volunteers, and the Reynolds Club became the institute’s headquarters. By the end of World War II, Chicago had trained more than 1,700 meteorologists.
The program’s early surge was driven by practical need, but it also created momentum to pair investigation with dissemination. The curriculum, which crammed two years of material into a nine-month course, included common wisdom from old meteorology, but it was grounded in fluid dynamics. “Rossby was very much in the philosophy of training the next generation of meteorologists based on physics,” says Noboru Nakamura, a professor in geophysical sciences who studies the jet stream—not simply how to read a weather map but how to analyze data (often collected by Air Force pilots) and recognize global patterns.
After the war ended, the institute, by then a full-fledged department, returned to its original focus on fundamental research, forming what would become known as the Chicago school of meteorology, with a heavy emphasis on physics, mathematics, and modeling. It was an exciting time rife with “untested theories that needed a proof of concept,” says Nakamura. Data were flying in, ready to be applied to theoretical equations to see if they held up in practice.
Guided by wartime-gathered observations, Rossby’s team revisited his planetary waves. After bombing missions in Japan, B-29 pilots reported winds as high as 230 miles per hour at cruising altitudes. The winds caused bombs to miss targets and, when positioned as headwinds, burned extra fuel, draining the aircraft. Rossby thought these winds must be associated with his high-altitude waves and developed a mathematical theory to predict their behavior. He named these fast-moving rivers of wind jet streams, and they are now used in both weather forecasting and aviation planning.
Meteorological research can be split into different scales of size. The Norwegians, who approached weather from a comprehensive point of view, prioritized large movements and trends over localized phenomena. Their polar air masses, as well as low-pressure cyclones and hurricanes, are called synoptic scale phenomena. Rossby went bigger; jet streams are part of global scale meteorology, incorporating models of circulation around the planet. But research at UChicago also went smaller, into the meso, or middle, scale. This subfield of meteorology includes thunderstorms and tornadoes, and it blossomed after the war.
A significant contribution to mesometeorology came from the Congress-mandated and funded Thunderstorm Project. A collaboration among the Weather Bureau, the Army Air Force, the Navy, and the National Advisory Committee for Aeronautics (NASA’s predecessor), the project studied storm causes and characteristics. Byers was appointed director. The project, which began almost immediately after the war ended, had access to airplanes, weather instruments, and an enormous cadre of trained personnel. It also had radar—previously highly classified technology used to monitor enemy aircraft—that was capable of tracking thunderstorms and visualizing precipitation structure within the clouds.
The Thunderstorm Project sent pilots through storms in radar-outfitted Black Widow warplanes, flying in a vertical stack at different altitudes to gather data on the storms’ traits and evolution. The data collection lasted a little over a year in two phases. Then UChicago scientists analyzed the massive data sets by hand in a two-year effort. The discoveries made during the Thunderstorm Project provided foundational knowledge for severe weather research: for example, the discovery and characterization of a storm’s three-stage life cycle.
During the time that Byers was leading a literal squadron of meteorologists into thunderstorms above Florida and Ohio, another man was climbing a mountain in Japan to collect his own data. They both arrived at similar conclusions about the nature of storms. Impressed that one individual with so few resources could achieve so much, Byers invited Tetsuya Theodore Fujita to Chicago, where he soon became director of the Mesometeorology Research Project. (For more on Fujita, see “Singing for the Pine Trees Are Stormy Winds.”)
Fujita went on to conduct his own wide-scale, data-driven investigations into tornadoes. His unconventional approach was long rejected by the meteorological community, says Nakamura, who counts Fujita as one of his heroes. “But our university has traditionally been nurturing to unconventional, creative approaches to science,” he says, “and supportive of the scientists doing work that may not have been possible elsewhere.”
In 1956, a year before he died, Rossby, then living in Stockholm, spoke at a meeting of the newly formed National Academy of Sciences Committee on Meteorology. He advocated for meteorologists to work closely with oceanographers, geographers, and geologists since the atmosphere affects and is affected by both land and sea. He called the atmosphere a “milieu” that influences all of human experience. Over the next few years, the expansion of meteorology into planetary science took hold across the field. In 1961 UChicago’s meteorology department merged with geology and became the Department of Geophysical Sciences.
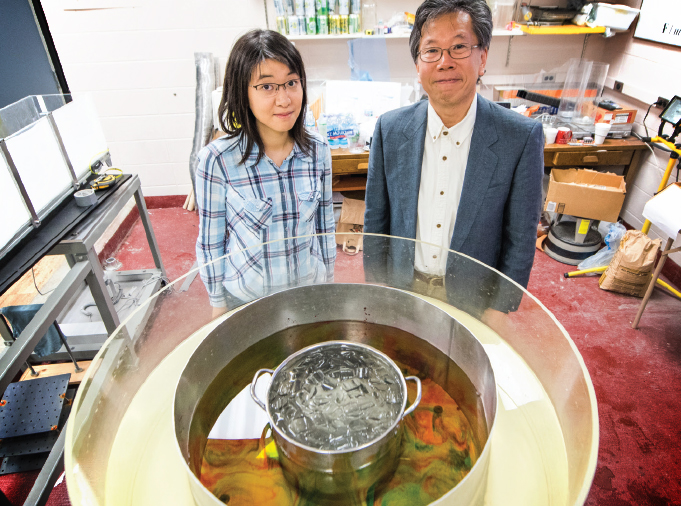
Today the department focuses on Earth’s atmosphere, oceans, glaciers, and climate; surface, interior, and evolution; and biosphere. Researchers in the department also look to space, studying exoplanets and cosmochemistry. Severe weather research has ceased.
“The vision of the department from the outset was to approach Earth science from the system point of view,” says Nakamura, “not just from individual disciplines but with a holistic view of Earth’s environment.” That vision was met with some resistance, he thinks, because different styles of inquiry were merged. “Meteorology was considered part of physics at the University of Chicago,” just as Rossby had originally inspired, “whereas geology was a completely different enterprise.”
Nakamura considers himself a “sort of sole survivor of that time,” arriving at UChicago in 1992. His recent research concerns how and why jet streams can get disrupted, and how that can lead to anomalous weather. He compares jet streams to highways that weather systems travel on, but they’re not straightaways—they meander. And large-scale features, such as mountains, land-sea transitions, or expansive land masses, change the streams’ internal dynamics, like changing the speed limit along a highway. At lower speeds, traffic can jam up. Nakamura uses fluid mechanics to explain why this happens, simplifying as much as possible, the way Rossby did with his waves. In doing so, he’s been able to draw mathematical connections to automobile traffic flow.
“I’m more interested in the short term,” says Nakamura, from a few days to a few weeks—the timescale for weather variability. Longer term analysis starts moving into climate science, which has a larger representation in the current geophysical sciences department.
Shaw’s climate work involves testing theories about the way the world works using numerical simulations as well as making observations to look for emergent patterns. “My research focuses on large-scale features, whether it’s Rossby waves or the jet stream or the Hadley circulation, which dominates how air moves in the tropics,” says Shaw. One question she seeks to answer is how such features respond to climate change.
Current models for forecasting future climate are sophisticated, taking into account complex scenarios. But for theoretical physicists, the questions always arise: What are the minimal physical ingredients required to explain emergent patterns in response to climate change? Can climate behavior be understood on a more fundamental level using simpler tools? “We have a big model with all the bells and whistles,” says Shaw, “and then we strip away the extra, leaving only the essential, the way Rossby did.”
Yet models eventually need to be applied to the real world, assumptions tested. Physicists can do so using atmospheric observations and then calibrating the models accordingly. Shaw fits into the bigger picture by “pushing the boundaries of the theories developed by Rossby and his colleagues by testing them on different climates,” using observations from both past and present. If the theories are sound, they should be able to explain the continuum of a changing climate. “And that’s how we build our confidence in future projections of Earth’s climate for the next hundred years.”
To learn about two meteorology students from the World War II era, see “A Change of Climate.”