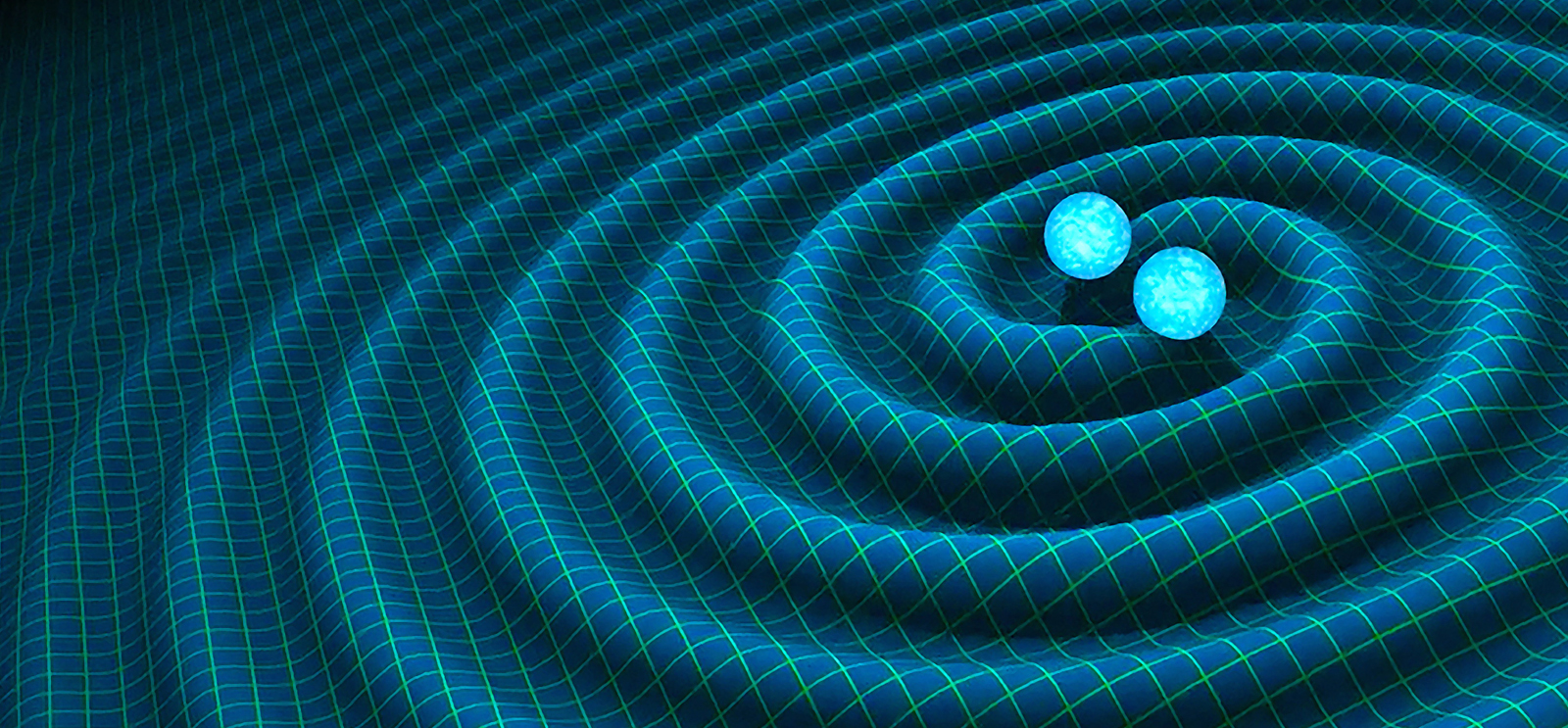
An artist’s impression of gravitational waves generated by binary neutron stars. (Illustration by R. Hurt/Caltech-JPL)
Albert Einstein predicted the existence of gravitational waves a century ago. Daniel Holz was part of the team of scientists that finally found them last fall.
Daniel Holz, SM’94, PhD’98, is an associate professor in the Department of Physics, the Department of Astronomy and Astrophysics, the Enrico Fermi Institute, the Kavli Institute for Cosmological Physics, and the College. He is also a member of the Laser Interferometer Gravitational-Wave Observatory (LIGO) collaboration, founded in 1997 to search for ripples in space-time, which would confirm a key part of Albert Einstein’s 1915 general theory of relativity.
In September LIGO detected these gravitational waves. The discovery not only confirms Einstein’s century-old theory but also marks the beginning of a new era in astrophysics. Holz spoke to the Magazine about what it’s like—and what it means—to hear two black holes colliding.—Maureen Searcy
I was in bed when I got the news. It was around 7:00 in the morning on September 14, and I was checking email before work. There was a message with the subject line, “Very interesting event.” I didn’t believe it, though. No one believed it. We test ourselves to make sure our equipment is working—there are people who secretly create fake events, and then we see if we can find them. We were in testing mode, so surely it was fake. But very quickly, within two and a half hours, we’d done preliminary analyses and it was fairly clear it was real. So by the time I got to work, I was already intrigued. By lunchtime, I was excited. By dinnertime, I was living the dream. It was real, it was happening, just like we had predicted. We had detected gravitational waves.
Einstein was right. In 1905 he developed his theory of special relativity, which, in addition to producing his equation E = mc2, defined space and time as one unified construct: a four-dimensional fabric of the universe. His theory of general relativity, published a decade later, uses the nature of space-time to explain gravity: it’s not a force but instead is the result of space-time warping around massive objects. Put a bowling ball at the center of a trampoline; the fabric will dip and wrap around its bulk. Now put a marble at the edge of the trampoline; it will roll toward the bowling ball.
The marble isn’t pulled by the bowling ball; it’s rolling down the curvature in the trampoline caused by the bowling ball—that’s gravity. Now bounce the bowling ball; it will send ripples through the trampoline, and that’s what gravitational waves are—ripples in the fabric of space-time. It’s not just a metaphor. If you shake an actual bowling ball around, you make gravitational waves, on a tiny scale. Whenever anything accelerates, gravitational waves propagate outward, letting the rest of the universe know that something has changed. These waves travel at the speed of light and are very weakly coupled, meaning they don’t interact much with matter, so they go through everything. We’re constantly bathed in these waves. But they are very difficult to detect, even when produced by a cataclysmic event.
For waves to be loud enough for us to hear, they must be generated from an extreme event, such as black holes or neutron stars smacking into each other at roughly light speed. The waves we detected in September were produced by two black holes crashing into each other a billion light-years away. This collision was phenomenally energetic, for a fraction of a second outshining the entire rest of the universe combined. And that was another part of Einstein’s theory, that there should be these mysterious regions of space-time with insurmountable gravity; everything falls in and can’t escape, including light. He called them singularities, which are at the center of what we now call black holes.
For a long time people thought they weren’t real—they were some extreme, mathematical thought experiment that we could never hope to prove and that probably didn’t exist in nature. We couldn’t directly observe them as they don’t emit light. We could only infer their existence by looking at their presumed effect on the surrounding universe, the way light bends and such. By detecting gravitational waves and measuring them, we’ve shown that black holes do in fact exist, and behave the way Einstein predicted. We can only detect waves if the event occurs reasonably close to us, though. A billion light-years is really close in this language, and within this distance we think these events happen many times a year. We just needed technology advanced enough to detect them.
I’ve been studying relativity since I was an undergrad. My junior year at Princeton, we were encouraged to do a research project. I approached a professor in the physics department, John Wheeler. He had done a lot of work on black holes, actually coined the name, and was one of the legendary figures in the theory of relativity. It was intimidating. I remember standing outside his door, too nervous to go in. But I had to find an adviser—it was getting late and I was desperate. I figured, what do I have to lose? So I went in, and that’s the moment everything shifted. That first time I introduced myself, he sat me down, and I was there over two hours. I walked out with a project—on gravitational waves and black holes, no less. For the next year and a half, I worked with Wheeler nonstop; I was in his office for many hours almost every single day. It was an incredible experience.
When I came to the University of Chicago for graduate school, my adviser was Robert Wald (Charles H. Swift Distinguished Service Professor, Department of Physics, Enrico Fermi Institute, and the College), who had been Wheeler’s student and is an expert on general relativity and black holes. I’ve been working on gravity ever since. I joined the Laser Interferometer Gravitational-Wave Observatory (LIGO) collaboration a couple of years ago. I had all these questions about LIGO. I had been writing theoretical papers about gravitational waves and black holes and neutron stars and cosmology. If I really wanted to make these a reality, I should try to learn from and contribute to the effort to detect gravitational waves. So I joined the collaboration.
LIGO is the most sensitive instrument ever built by mankind. It consists of twin detectors, one in Livingston, Louisiana, and the other in Hanford, Washington. These devices act like antennae to detect gravitational waves, using lasers to register even the slightest “wiggle” in space-time. If both detectors, separated by thousands of miles, wiggle in a predictable way at about the same time, we know it’s a wave event and not caused by some local disturbance. When LIGO was proposed in the early ’90s, people thought it was crazy, that taxpayer money shouldn’t fund it because it was impossible. The instrument can’t possibly be sensitive enough. And if it is, what if gravitational waves aren’t even real? Or what if there are no sources of gravitational waves loud enough for us to hear?
But LIGO was built, and it works. We could have been waiting a long time to find out if it worked because we weren’t sure how frequently these powerful events happened. I coauthored a series of papers saying they should be fairly common, that we’d see an event maybe once a month or so. We found something just a few days after turning on. One resounding detection. Gravitational waves aren’t sound waves—they’re not compression waves in the air—but they have similar properties. You can take the gravitational waveform and put it directly into speakers. So we did that, and it sounds like a thump. You can hear it with your unaided ear, even with the noise. We expected most of the sources to be very difficult to hear, but this one was really loud. That’s part of the reason we’re so confident. It’s beautiful and perfect.
The LIGO collaboration is enormous. The discovery paper, published in February, has 1,004 coauthors. The UChicago contingent includes myself; Ben Farr, a postdoctoral McCormick Fellow in the Enrico Fermi Institute; astronomy graduate student Hsin-Yu Chen; and physics graduate student Zoheyr Doctor. Part of the research we do involves deciphering the waveforms. We detect something, and then we ask, well, what was that? You have to turn the data into a statement: that was two black holes, each 30 times the mass of the sun, merging a billion light-years away in that part of the sky. We help figure that out.
Now that we’ve detected gravitational waves, now that we know they’re real, we can start probing questions about the evolution of the universe, how stars live and die, how black holes form. This discovery opens the door to so much astrophysical and cosmological exploration. This feeling is hard to describe. It’s something I’ve been working on essentially my entire career. You spend all this time searching for something, and then suddenly you hear it; the event is in your data, loud and clear. It’s a moving experience. Even now, it gives me goose bumps to think about it. I see a plot of our results, and I just can’t help but smile.—Daniel Holz, SM’94, PhD’98