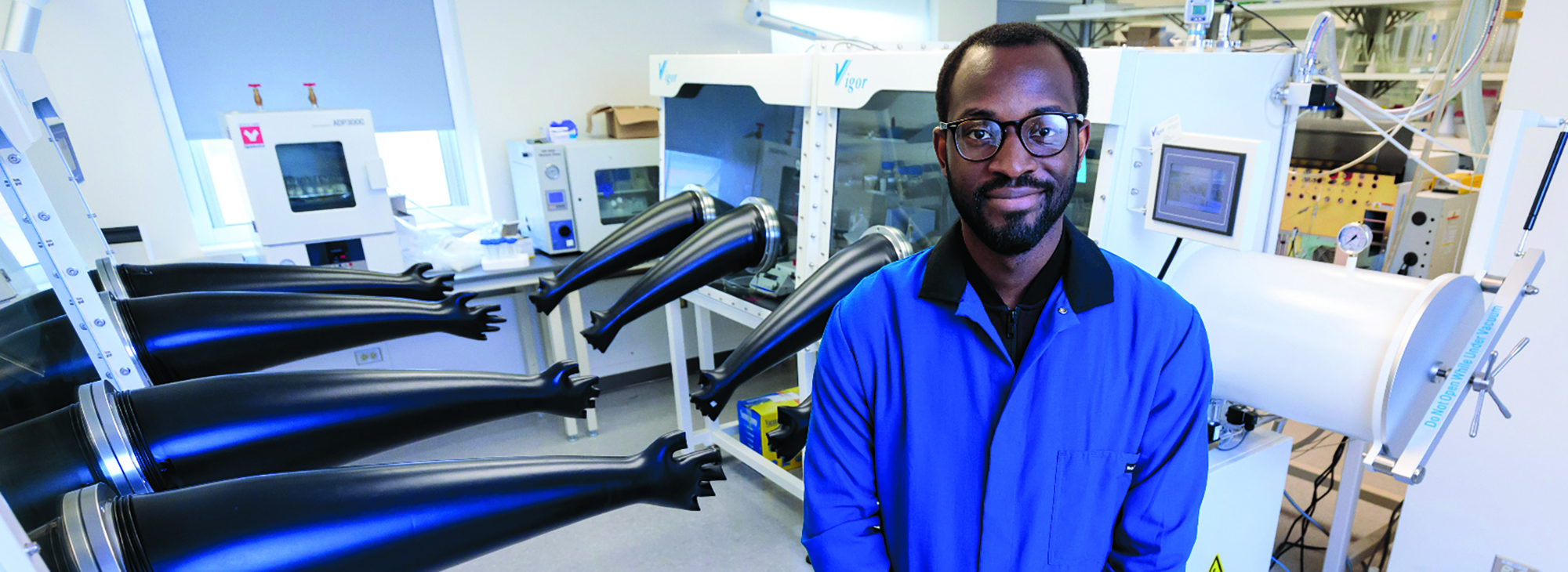
Chibueze Amanchukwu, who joined the UChicago faculty as a Neubauer Family Assistant Professor of Molecular Engineering in 2020, is working to improve battery technology—with an eye toward combating climate change.
Chibueze Amanchukwu seeks a chemical key to sustainability.
In April UChicago molecular engineer Chibueze Amanchukwu had the opportunity to testify at a congressional hearing on sustainable energy. The takeaway: the government must continue funding basic research into batteries, which should be built in the United States with materials sourced domestically. “One thing that the pandemic has shown us,” he says, “is how fragile supply chains are.”
An immigrant from Nigeria who first arrived in the US as a high schooler, Amanchukwu was offering policy advice to his adopted country for good reason. With Earth’s atmosphere straining under an estimated trillion tons of carbon dioxide, renewable energy sources like wind and solar need to scale significantly—and quickly. And this will require upgrading a technology that Amanchukwu knows a lot about: batteries.
“You don’t always have the sun shining or the wind blowing,” he says. Because these renewable energy sources are intermittent, their ability to replace fossil fuels is limited by the ability of batteries to store energy in the downtimes.
Battery technology is a bottleneck of climate action.
Amanchukwu’s road to working on this problem started when he was a child. If you do well in school in Nigeria, he says, you are expected to become a doctor, lawyer, or engineer. “I liked math, I liked chemistry,” he says, “and I also knew you could make a living doing that.” When he came to America as a 10th grader, he quickly found himself busy thinking about applying to colleges. “But it was exciting. There was this whole new world of opportunities.”
After majoring in chemical engineering at Texas A&M and earning his PhD at MIT, he joined the UChicago faculty as a Neubauer Family Assistant Professor of Molecular Engineering in 2020, with a joint appointment at Argonne National Laboratory. He says that coming to the Pritzker School of Molecular Engineering was a “no-brainer” because of its alignment with his research objectives and the exceptional quality of its scientists.
Today Amanchukwu’s lab is working to address what he sees as a twofold problem. First, battery lifetimes need to improve to suit the intense demands of electric transportation; second, batteries must become cheaper—cheap enough, he says, “to be deployed on the scale that we need to power cities and nations.”
Batteries have three main components: two electrodes (an anode and a cathode) and an electrolyte. The electrolyte, typically composed of a salt dissolved in a liquid, is the medium that separates the electrodes. When electrons are released from the anode, they leave behind positively charged ions, which travel through the electrolyte to the cathode. Among these three, Amanchukwu’s attention is squarely on electrolytes.
“Currently the best way we make electrolytes is through trial and error,” he says. But of all the billions of compounds you could put in an electrolyte, how do you know which ones will lead to the most promising and least troubling properties?
The question is pressing because electrolytes are a clear area for improvement in battery technology. For instance, today’s standard rechargeable battery, the lithium ion, relies on a flammable electrolyte that can lead to explosions, while the battery loses significant capacity after about a thousand charges. The formation of disruptive metallic microstructures called dendrites has also been a problem in some lithium-based batteries.
Machine learning may be the key to electrolyte design. Amanchukwu and his lab are training a machine-learning model to help answer an important question: Given a certain chemical compound, what properties will it exhibit as an electrolyte? Answering this question up front means weeding out unpromising compounds before investing time and money in them.
Amanchukwu is even more interested in the inverse question: Given a desired electrolyte property, which compounds will have it? For example, if you know that a car battery needs to be within a certain voltage range, could the computer model tell you which compounds can pull this off?
Answering these questions requires data. The second question is particularly daunting, since the lab is training its algorithm with previously published electrolyte data—which is limited, Amanchukwu says, by the literature’s focus on a type of chemical that will not pass muster for next-generation batteries. “With all the data skewed toward one specific chemical structure,” he asks, “is the model able to predict a new class of molecules that haven’t been synthesized before?” The answer is unclear.
Amanchukwu’s colleagues recognize the promise of his work. Nanomaterials specialist Junhong Chen, the Crown Family Professor of Molecular Engineering, says, “Amanchukwu’s research on electrolytes could potentially transform various electrochemical processes, ... all of which will accelerate our decarbonization efforts.”
“Decarbonization” means not just reducing emissions but removing carbon that is already out there. Carbon capture technology currently relies on one electrolyte to dissolve CO2 from the air and another to convert it into a valuable product (such as the chemical ethylene, which is vital for manufacturing). Amanchukwu hopes to increase the efficiency of this process—and the commercial viability of the resulting products—by designing an electrolyte that can combine these two steps.
In both battery design and carbon capture technology, Amanchukwu knows he is working on solutions that are in high demand. “If you develop a new battery today that is cheap enough, that is energy-dense enough, it will get deployed tomorrow—the world is hungry.”